One
of the most interesting features of metallatranes, first prepared back in
60's, is the intramolecular dative coordination N->M
(M = Si, Ge, Sn) which accounts for their specific properties in various
contexts. We undertook first study on electrochemistry of silatranes and
germatranes with 5- and 6-membered lateral chains, either purely aliphatic or
containing fused aromatic rings.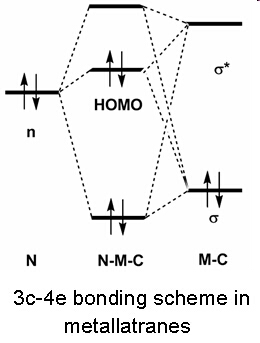
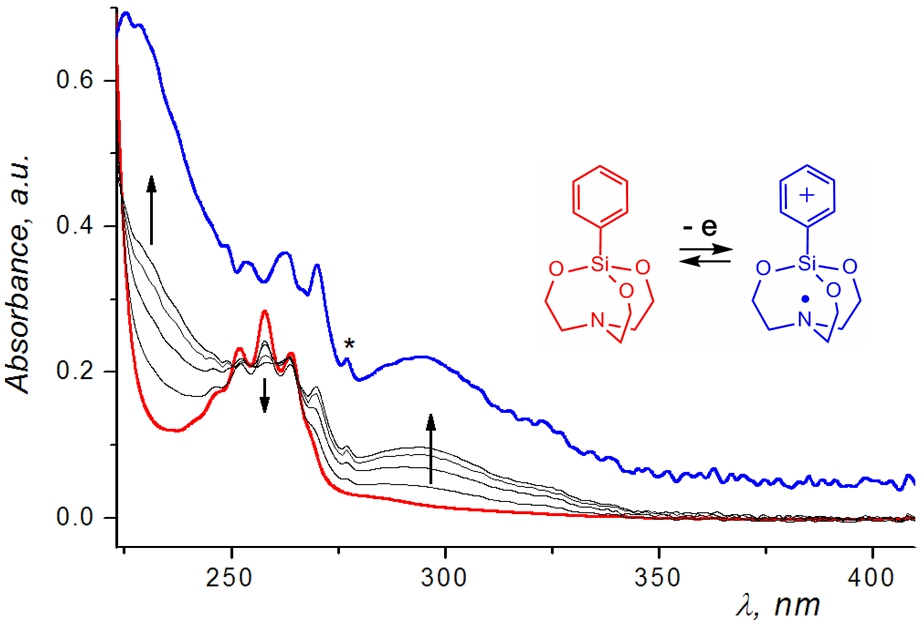
Though
the lone pair of nitrogen is largely involved in the 3c-4e N->M
coordination, electrochemical electron transfer (ET) is reversible for all
metallatranes studies, unlike tertiary amines; the UV spectra of their cation
radicals show new absorbance band due to SOMO-LUMO transition.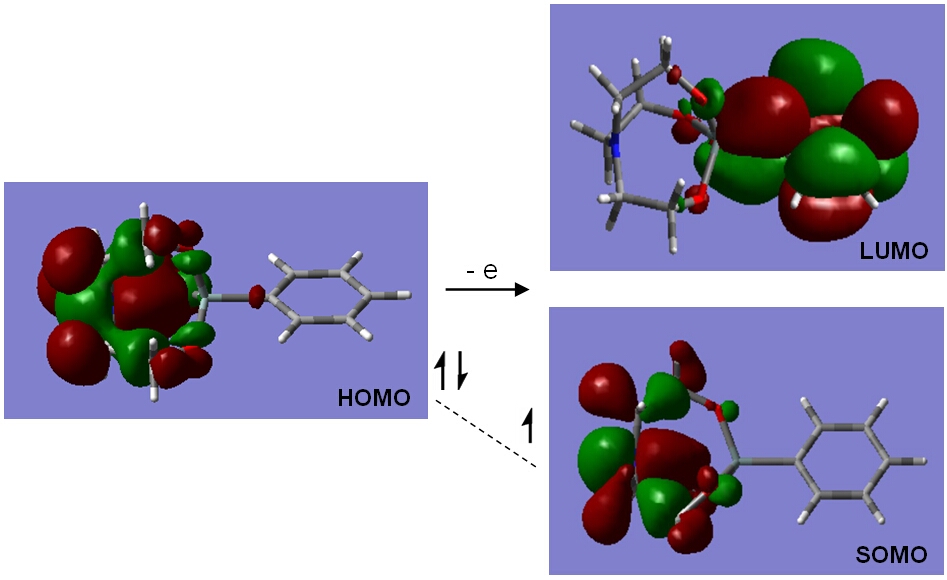
When lateral conjugation of the M-C part of the N-M-C 3c-4e bond is impossible
because of orthogonal orientation of the p-type
orbitals of the substituent R (Ar, Npth, vinyl etc), distonic cation radicals
are formed, with the spin remaining at nitrogen and the positive charge
distributed over M and the lateral branches of the atrane cage.
EPR-spectroelectrochemistry reveals large
(end-to-end width D
~
160 G) well resolved spectra organized in 9 groups with relative
intensities as 1:1:4:3:6:3:4:1:1, as e.g.
for Ph-silatrane
cation radical.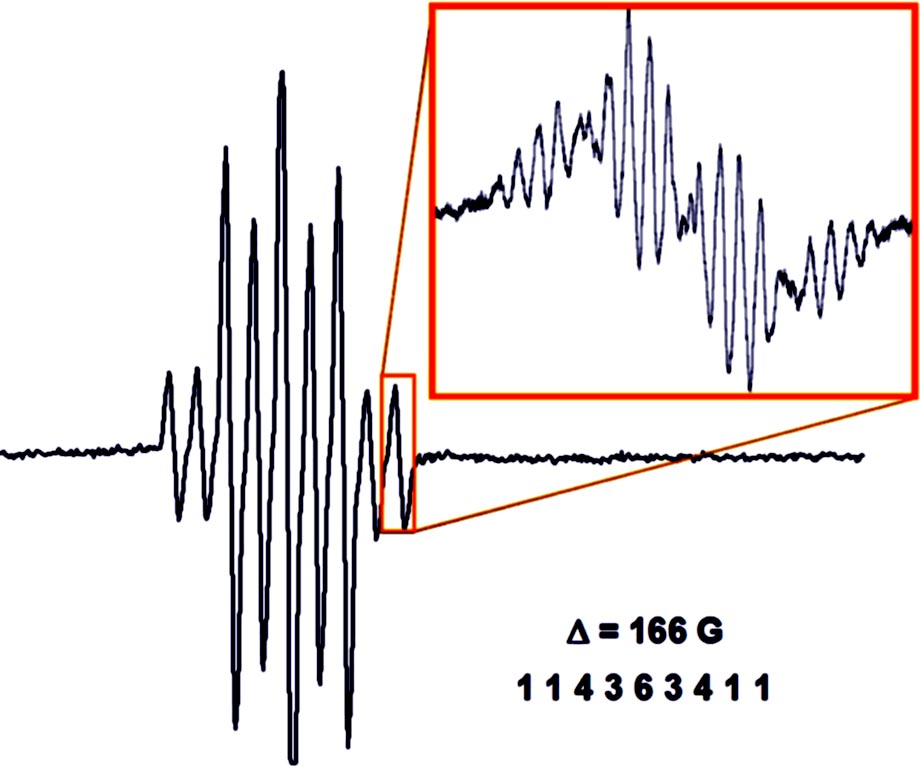
There follows that N atom is practically planar (hfc
aN = 18-19 G, NBO
electronic configuration of N is 2s(0.69) 2p(2.4) and N(pz) NAO
occupancy is 0.985) and three a-C-H
bonds are axially oriented showing most conjugation with the spin carrying N(pz)
orbital. Both
ESR data and Fermi contact couplings from DFT B3LYP/LANL2DZ
show no contribution from M in the spin delocalization.
The geometry changes around M in these cation radicals show opposite trends:
Si becomes closer to trigonal bipyramid geometry, Ge
atom can go into or out of Ge(-O)3 plane preferring trigonal
bipyramid
or tetrahedral configuration, respectively, while Sn mostly flattens as
follows from ESR data and DFT B3LYP/LANL2DZ calculations.
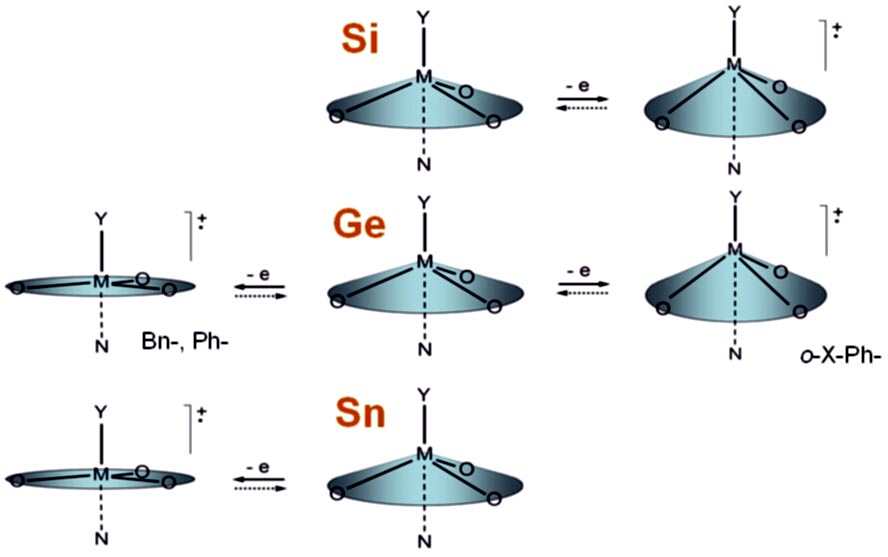
Even when R has lower own IP than N of the atrane (Alk2NC6H4-,
thienyl etc) and the atrane moiety is not immediately affected by ET, thus
induced positive charge on M markedly changes its geometry.
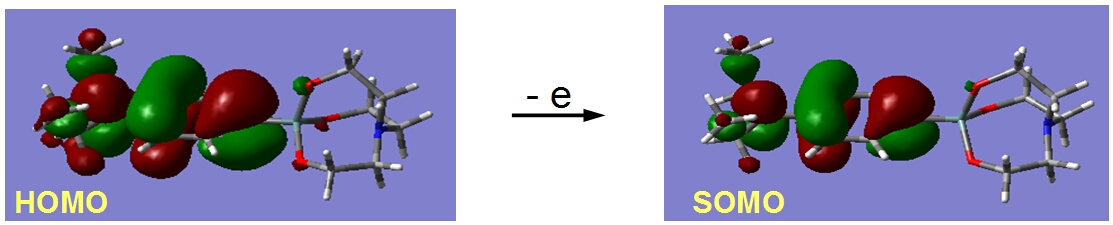
In contrast to aryl- and vinyl-substituted metallatranes, hyperconjugation of s(Si-Csp3)
bond in the cation radicals of benzyl germatranes enables lateral "spin
leakage" from N to the benzylic aromatic ring of the substituent. The
formation of distonic cation radicals of metallatranes with the R groups
"ending" electron transmission and electrochemically induced
geometry changes around M atom will provide a new insight into the electronic
interactions in these compounds and open possibilities for designing molecular
electronic devices with specific electronic, optical and electromechanical
properties.
ESR-spectroelectrochemistry also enabled us to make evident the process of penta-
to hexa-coordination changes in the cation radicals of arylgermatranes.
Primary cation radicals of o-Br and o-F-Ph germatranes are formed in the
geometry close to that of the starting molecule and then slowly evolve to
adopt 6-coordinated configuration with additional coordination from ortho-halogen
substituent. Kinetics and activation parameters of this process were measured
as well.
return